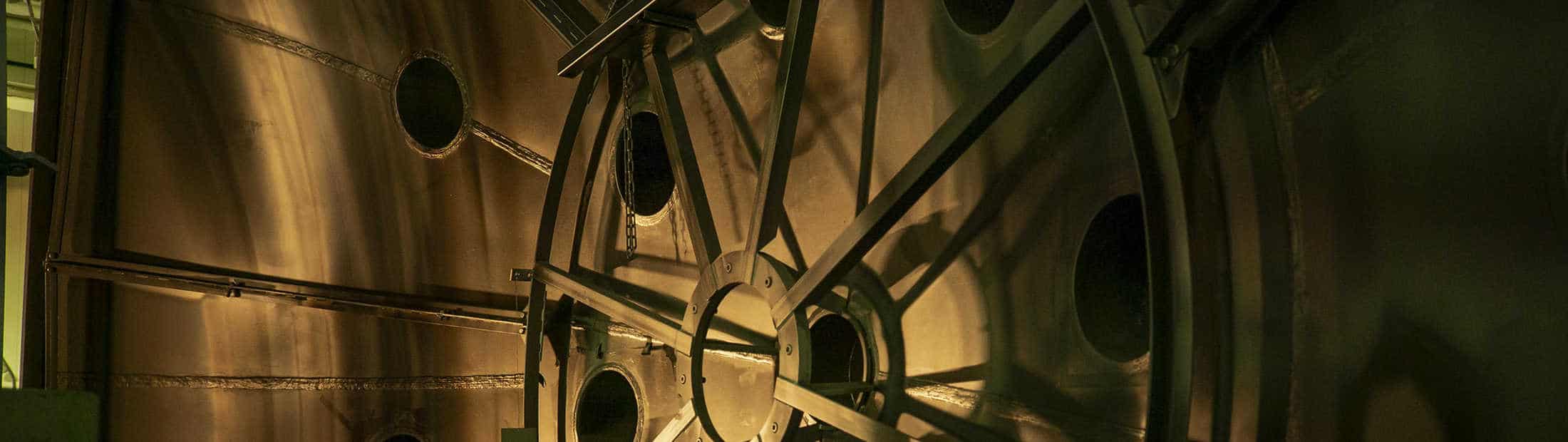
To the Moon and beyond: How University of Michigan Aerospace Engineering is on the cutting edge of electric space propulsion
The University of Michigan Aerospace Engineering Department’s Plasmadynamics and Electric Propulsion Laboratory (PEPL) is developing the next-generation of Hall thruster technology that may one day propel the first human missions to Mars.
It’s the year 2037 and in lunar orbit, near NASA’s Gateway space station, the first crewed mission to Mars is ready to depart. In the command module, the tension is high as the six astronauts sit strapped in their couches. On the large forward monitor screen the countdown for the engines firing ticks down dramatically. Five… Four… Three… Two… One…
And nothing. No jetlike roar, no vibrations, no crushing acceleration. A few lights go from red to green, the pilot’s display switches to flight mode, and there doesn’t seem to any sense that the spacecraft is moving. Meanwhile, a video feed from a CubeSat recording the historic launch show not a huge bell-shaped rocket belching fire on the stern of the craft, but what looks like a row of fluorescent lamps shaped like targets with glowing concentric rings nested inside one another as the Mars ship seems to hang motionless in space.
Has something gone wrong? Quite the opposite. In fact, the Mars mission is well on its way as it is pushed out of orbit by the latest generation of Nested Hall Thrusters (NHT) that produce only a few pounds of thrust, but can fire for months or even years on end, allowing them to eventually move spacecraft at tremendous velocities with much more efficiency than conventional chemical rockets.
This may sound like Star Trek or Star Wars, but smaller versions of these engines and variants of them are very real, very much based in the established physics of today, and have been flying on space missions in surprising numbers for decades. The Hall thruster is a technology that the University of Michigan Department of Aerospace Engineering is playing a leading role in developing and is a key component in NASA’s plans for its next 20 years of space exploration.
Put simply, a Hall thruster is a form of electric space propulsion that replaces conventional rockets with a stream of charged ions that can propel a spacecraft for months or years on very little fuel while being powered by an outside source, like an array of solar panels, a nuclear reactor, or fuel cells. Electric Propulsion a technology that’s been used before, notably on NASA’s Dawn asteroid-hopping mission, but Michigan is improving on the existing state of the art by an order of magnitude that could be compared to the jump from subsonic to supersonic air travel.

The latest version developed by UM carries the science-fictional name of X3. It’s not only the biggest Hall thruster yet, but has broken records for the maximum power output, thrust, and operating current achieved by any Hall thruster to date. This throttleable engine can run at a power range from 5 to 102 kW and generate 5.4 Newtons or about a pound of thrust (the equivalent to the weight of a book). That’s three times more than previous thrusters and all while measuring only about a meter wide and weighing in at 227 kg (500 lb).
One of the leading figures behind the X3 project is Dr. Benjamin Jorns, an Assistant Professor at the University of Michigan Department of Aerospace Engineering and Co-Director of UM’s Plasmadynamics and Electric Propulsion Laboratory (PEPL) – founded by Alec Gallimore and the second oldest plasma research laboratory in the United States.
What I propose is not physically real. It violates some of the tenets of General Relativity. If you want to do advanced propulsion, do electric propulsion. And that’s how I got into it.
Benjamin Jorns, Professor of Aerospace Engineering
Previously a member of the space electric propulsion group at NASA’s Jet Propulsion Laboratory (JPL) in Pasadena, California, Jorns has long had a desire to find a way to get humanity out to the stars.
“To me, there was something patently unfair that things were so far away in space, so when I was younger I wanted to work on things that made it easier to get around in space,” says Jorns. “That led to propulsion. When I first started looking at this in high school, I said ‘I want to work on the warp drive’ and at the time, there was a popular science article by a physicist who had written a paper about how the warp drive was theoretically possible. I wrote him an email and he wrote me back and he said, ‘What I propose is not physically real. It violates some of the tenets of General Relativity. If you want to do advanced propulsion, do electric propulsion. And that’s how I got into it.”
Jorns and the UM aerospace team are working on not only increasing the size of Hall thrusters but also on turning them into a practical system that may one day propel crewed missions to the Red Planet. This is not just a conjecture about some maybe future, but part of NASA’s long-term plan to put American astronauts on Mars.
NASA’s NextSTEP program, which ended in 2019, funded several propulsion systems for a crewed Mars mission and one of these is the XR-100 Hall thruster. This was developed by Aerojet Rocketdyne in collaboration with NASA’s Glenn Research Center, the University of Michigan, and the Jet Propulsion Laboratory in Pasadena, California. The goal was to come up with a next-generation, high-power Solar-Electric Propulsion system (SEP) that can operate at levels above 100 kW – enough, when more than one is used, to move large-scale cargoes for crewed missions to the Moon and Mars.
X3 is UM’s lab prototype that may one day be the basis for the XR-100. It may not be warp drive, but Jorns says that Hall thrusters like the X3 are to conventional rockets what electric cars are to cars powered by internal combustion engines – making the X3 the Tesla of spaceflight.
This is because the rockets that lift off so spectacularly from places like Cape Canaveral in Florida run on liquid fuels like kerosene or liquid hydrogen that are burned with liquid oxygen or a chemical oxidizer. These can generate enough thrust to hurl a robotic probe clear out into the Solar System, but this thrust is limited by the fact that the energy to propel the rocket comes from the breaking of chemical bonds. In other words, the propellant that pushes the rocket is also the fuel that releases the energy to do the pushing.
What this boils down to is that a chemical rocket can produce tremendous thrust, but only for a very short time usually counted in minutes, which limits the payload compared to the fuel needed to lift it. Worse, such rocket engines are very complex affairs with many parts and intricate systems to both feed fuel to the engine and to keep it from melting from the tremendous heat generated.
The X3, on the other hand, doesn’t have the familiar rocket nozzle and doesn’t burn its propellant with an oxidizer. Instead, it works by taking heavy gas atoms like xenon or krypton and inject them into a cylindrical chamber through an anode that strips them of an electron, turning them into positively charged ions. In the chamber, a magnetic field is used to capture and hold electrons in a cloud of spiraling orbits at the chamber’s open end, which acts with the cathode to draw the ions to it, accelerating them until they are ejected into space at very high speeds of up to 20 times the exhaust velocity of a chemical rocket.
Though the xenon atoms are moving very fast, the thrust they produce is tiny in comparison with a chemical rocket, but the X3 can fire for much longer and is far more fuel-efficient, meaning a spacecraft can get a much higher velocity for each unit of propellant carried aboard.
The basic principle has been known for over a century and has been used in smaller, less powerful thrusters that have been flying in space since the 1960s. Today, hundreds of SEP systems have been launched with most of them being used for attitude control on satellites and they have even been used on NASA’s Dawn asteroid-hopping mission, where the engine ran for seven years.
What makes the X3 stand out is that UM has been focusing on how to scale up the design. Put it another way, how to get the power needed for something really big like a Mars mission? The X3 can’t just have a higher thrust than previous engines. It also needs to be robust, easy to build, and scalable, allowing for small engines as well as very big ones. To give an idea of the importance of scale, Dr Jorns says that the current generation of electric propulsion could take decades to spiral a crewed mission to Mars while something like the X3 would take 2-3 years. The goal is to one day produce a Hall thruster that can do it in months.
X3’s answer is called channel nesting. That is, instead of making one big engine or a lot of little engines, the X3 is made of a series of concentric rings nested inside one another to form three channels that can be powered up individually or in any of seven configurations desired without the need for lots of duplicate systems. Dr Jorns says that this design may be a very good payoff with an 80 cm (31 in) engine being the equivalent to one of 150 cm (59 in) in diameter and much lighter.
The descendants of the X3 and similar Hall thrusters have the promise of playing a key role in NASA’s plans for the next 20 years. Four thrusters are already planned to be installed on the space agency’s Gateway crewed outpost that will be placed in cislunar orbit, where it will act as a forward base for lunar exploration and crewed Mars missions – with the latter becoming feasible when thrusters that can operate at 600 to 800 kW in clusters are available, backed by chemical rockets giving additional boosts as needed during the flight.
In addition, such thrusters could also be used for asteroid mining, servicing satellites that need refueling or repair, and disposing of dead satellites before they become a hazard to other spacecraft. And, if history is a guide, the more powerful the engines become, the more applications will present themselves.
One way would be to go with clustering small engines and just install a lot of them to get the thrust you want. That’s fine for a small number, like a half dozen, but things add up quickly and that means not only lots of engines but lots of pipes, pumps, electric cables, sensors, and so forth, which means a lot more weight. Worse, every engine added means the odds of something going wrong multiply very quickly.
Another way is to make bigger engines but this has its own problems – not the least of which is getting a giant thruster into orbit atop a rocket with limited payload space.

X3’s answer is called channel nesting. That is, instead of making one big engine or a lot of little engines, the X3 is made of a series of concentric rings nested inside one another to form three channels that can be powered up individually or in any of seven configurations desired without the need for lots of duplicate systems. Dr Jorns says that this design may be a very good payoff with an 80 cm (31 in) engine being the equivalent to one of 150 cm (59 in) in diameter and much lighter.
The descendants of the X3 and similar Hall thrusters have the promise of playing a key role in NASA’s plans for the next 20 years. Four thrusters are already planned to be installed on the space agency’s Gateway crewed outpost that will be placed in cislunar orbit, where it will act as a forward base for lunar exploration and crewed Mars missions – with the latter becoming feasible when thrusters that can operate at 600 to 800 kW in clusters are available, backed by chemical rockets giving additional boosts as needed during the flight.
In addition, such thrusters could also be used for asteroid mining, servicing satellites that need refueling or repair, and disposing of dead satellites before they become a hazard to other spacecraft. And, if history is a guide, the more powerful the engines become, the more applications will present themselves.
But there are still a lot of challenges to be met before anyone is packing their bags for Mars. For one thing, there’s much more to an SEP than the engine itself. There are also all the systems to run them. Hall thrusters need power and the big ones need massive solar arrays like those on the ISS to do the job. But those are too large and complex and they eat up the advantages of the thrusters with their mass, so the arrays need to be made more compact, lighter, and able to be launched as a single payload.
Then there are the electrical packages to convert and handle the power from the arrays and batteries for backup. These, too, need to be made smaller and lighter. This doesn’t even touch on all the pumps and plumbing needed to fuel the engines with propellant that need to be designed, built, and tested.
But according to Jorns, the main challenge is in testing the X3 itself and those that come after it. Live test firing of small engines that run for only a short time is fine, but when the high-powered jobs need testing, things quickly hit a bottleneck.
UM, with its Large Vacuum Testing Facility, has one of the best academic vacuum chambers in the world with one of the highest pumping speeds that combines low pressure with high-flow rate thanks to 19 cryogenic pumps. This is very important because once the engine gets past a certain power level because it is extremely difficult to recreate the vacuum of space and the X3 is definitely a denizen of space and space alone. On Earth, it would be a piece of scrap.

Worse, an engine designed to run for years can’t be allowed to monopolize one of these rare, expensive chambers for all that time, much less the 50 percent safety margin that engineers prefer. It simply isn’t economically feasible
This is the reason why UM is making such a close study of the X3. The goal is to gain enough data and enough of an understanding of the behavior and physics of the engine so that computer models can be developed. Such models could be run quickly many times to simulate many full missions, producing statistical models of the engine’s performance, allowing engineers to predict when and where it might fail, as well as how to head off and counter these failures before they happen.
All of this means a lot of time crunching numbers and working in a world populated by obscure phenomena, complex formulae, and scientific jargon. But the result may well be one day images sent back showing the first human footprint on Mars.
“I don’t know when we will see the first human will step on Mars, but we will only get there if we keep looking to the horizon, researching and developing the next generation of technologies,” said Dr. Jorns. “UM is playing a lead role in pushing this innovation, taking risks and trying new things to try to reach this end goal.”